Dental Anthropology – An Overview
DENTAL ANATOMY AND MARKS OF DEPOSITION
Teeth are comprised of four tissues: Enamel, dentine, cement and pulp. Enamel is the hardest substance present in the human body, consisting mainly (97% w/w*[1]) of calcium hydroapatite. Dentine presents a higher capability of remodelling than enamel, since it contains only about 70% w/w of hydroxyapatite crystals. The pulp contains blood vessels and nerves which supply the teeth and the cementum, with the periodontal ligament, anchors the roots in the sockets of upper and lower jaws.
Because there is no remodelling of enamel after its maturation, which usually occurs after 24 hours after the initial matrix secretion of ameloblasts, its original marks of deposition are retained as cross striations and brown stria of Retzius. Cross striations, also known as short period markers, arise through the circadian rhythm (represented by bands of different levels of mineralization deposited within a single day). The most plausible hypothesis for this pattern of deposition was suggested by Boyde (1964), and further developed more recently by Risnes (1998). They suggested that there would be more carbon dioxide available for incorporation of the enamel during periods of greater metabolic activity, causing enamel to be deposited faster during these moments compared to periods of lesser metabolic activity, which also explains the variation in mineral density found in these markers. The brown stria of Retzius represents a circaseptan cycle, which varies between 7 and 11 days between individuals, but it does not vary throughout the life of a single individual (FitzGerald, 1998). The origin of this cycle is not totally understood. Some authors hypothesise that it is under regulation of melatonin (Hasting and Loudon, 2006), others suggest that it may be a mere stochastic process (FitzGerald, 1995).
Human permanent incisors have about 150 brown stria, canines about 180 and molar contain 120-150 (Bullion, 1987), and the distance between each striae increases towards the incisal or occlusal surface. The wave-like pattern was termed perikymata, subdivided into perikyma grooves and ridges. Counting these markers provides a reliable method of estimating the age of subadults, as tooth eruption follows a strict biological sequence, with a well-known variance between populations. Disruptions of enamel deposition are usually due to genetic enamel defects (collectively referred as amelogenesis imperfecta) or more commonly caused by environmental stress (dietary deficiency, fevers, infections), refered to as hypoplasias.
EVOLUTION IN THE FIELD
Dental morphology plays a key role in multiple aspects of evolution because the dentition is under forceful genetic control. An animal with faulty dentition cannot properly breakdown food in the first stage of digestion, which leads to poorer absorption of energy and ultimately a reduction in the likelihood of its genes being passed onto the next generation.
In their origin, teeth were simple, one-cusped (haplodont), uniform (homodont), conical-shaped structures. Adaptation led to the development of more sophisticated structures, reflecting the versatile dietary aspects of a species. Mammals have heterodont teeth, represented by incisors, canines, premolars and molars: These created occlusal surfaces suitable to a greater variety of food (Phulari, 2013). Herbivorous mammals have molarized premolars, which enhance the surface area and optimize grinding of plant material. Carnivores have dicing/slicing specialized dentition with blade like posterior teeth called carnassials and molars reduced in size or even lost in some species (Hilson, 1986). Omnivores have a more generalized dentition.
Mammals evolved from reptiles in the Mesozoic era, and early mammals presented characteristic triangular-shaped molars (Hillson, 2005). Osborn (1907) hypothesized that the lingual cusp of upper molars and the buccal cusp of lower molars were homologous to the reptilian cone and called these the protocone and the protoconid, respectively. Two other cusps originated as cuspules, and via selective pressure developed into larger structures called the paracone and paraconid at the mesial end of the molar and metacone and metaconid at the distal end in upper and lower molars, respectively. This cusp triad is known as the trigon in upper molars and trigonid in lower molars. In most mammals, the paraconid has been lost in lower molars. An additional ledge of cingulum is present at the distal end of the trigon in primates, known as the talon in upper molars, and the talonid in lower molars. On the upper molars the talon houses a single cusp, the hypocone, but three additional cusps are found on the primate trigonid: hypoconid, entoconid and hypoconulid (Figure 1).
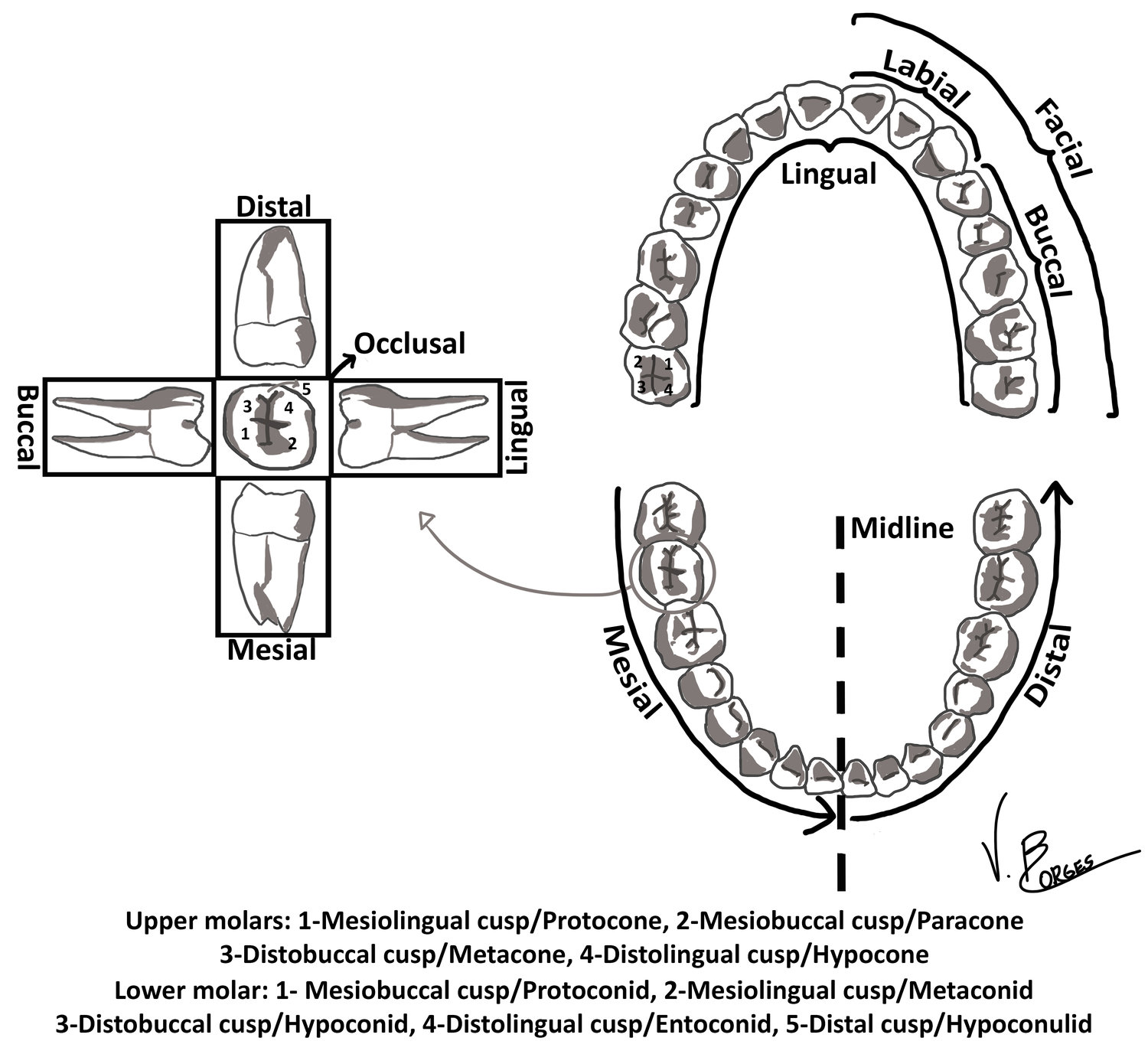
It is now known that the cusp homologous to the reptilian cone in upper molars is the paracone, not the protocone (Butler, 1978), as it was initially suggested by Osborn. Nevertheless, his original nomenclature is still used by most authors, although some prefer to use this terminology and describe the paracone as the primary cusp or cusp 1 (Steele and Bramblett, 1988). Trying to develop a clearer system, Hershkovitz (1978) created an entirely new terminology, replacing the paracone and the protoconid for the eocone and the eoconid. As described by Scott and Turner II (1997), while Hershkovitz has a strong case on paleontological grounds, Osborn’s terminology has such a lengthy history of usage that changing it would create more, rather than less, confusion.
A reduction in the number of teeth and generations of teeth is observed in recent species, possibly as an energetic trade-off for an increase in morphological complexity. Mammals originally presented three incisors in each quadrant of both maxillary and mandibular teeth. The majority of early primates lost one incisor, and by the Eocene there was a reduction in the number of premolars (Swindler, 2004). The modern human dental formula, shared by all catarrhine primates, is 2-1-2-3, while platyrrhines dental formula is either 2-1-3-3 or 2-1-3-2.
How diet influences dental and masticatory morphology is exemplified in early hominins. Paranthropus presents a more robust mandible, larger temporalis and masseter muscles, and larger posterior teeth with extremely thick enamel when compared to early Homo (Figure 2). This suggests that hard material such as nuts and seeds were an important component of the diet of Paranthropus (Grine and Martin, 1988). Some authors try to relate the trend of reduction of molar size, enamel thickness and masticatory muscles throughout the evolution of the genus Homo to factors such as meat eating (Bunn et al, 2012), the control of fire and cooking (Wrangham, 2009), or even tool use (Teaford, 2007).
EVOLUTION AS A FIELD
Fossilization is an extremely complex and ultimately rare phenomenon, which occurs under very specific circumstances. As a result the fossil record presents very few complete specimens of our distant ancestors. Enamel, the ‘inorganic coating’ of teeth, is the main reason teeth preserve so well in most taphonomic contexts and therefore a high percentage of the fossil record is made up of teeth. Even though other osteological elements are more informative in some respects, they are less likely to be preserved, so palaeontologists and paleoarcheologists have to work with teeth and dental traits in most contexts.
The precise description of dental traits is important, but not easy. Without a standard reference showing variability in the occurrence of dental traits, dichotomized nominal measurements, characterised by absence/presence, were used for many years in the field. In 1920, Ales Hrdlička published the famous ‘Shovel-shaped teeth’, which provided a set of ordinal measurements for the shovelling trait, which included the absence of the trait and three degrees of expression. Other workers had discussed morphological gradations in earlier papers (Black, 1902), but as criticised by Hrdlička, they did not focus on specific trait variance.
Although it is arguable that interval measurements from quantitative or metric data provide precise information with a lesser degree of error, the trend of non-metric observations has prevailed in the field. As described by Scott and Turner II (1997), most dental traits are not easily described by quantitative data, because: ‘(1) they exhibit variation in both form and size, not just size; (2) as three dimensional objects, they often lack the kinds of landmarks that are needed to standardize measurements; and (3) they may show such slight levels of expression, there is no way to measure them in millimetres.’
Basically, dental traits are not metric/non-metric per se, but the absence of precise tools and methods makes the description of most dental characteristics more attainable using qualitative rather than quantitative descriptions. Hanihara (1970) presented a creative way of measuring an ‘essentially qualitative’ trait (hypocone) through quantitative measures. He photographed of the occlusal surface of the molar, printed an enlarged version and cut the area delimited by the hypocone, which he weighed using a high-precision scale in order to stablish a relative area of the hypocone in relation to the total crown area, which created incredibly robust results. This technique has been since used by many workers (Yamada and Brown, 1988; Bailey et al, 2004; Benazzi et al, 2011).
Since Hrdlička there have been a lot of improvements in this field. Dahlberg (1956) and Hanihara (1961) were the first to develop a dental trait scoring system with plaster plaque references for permanent and deciduous dentition, respectively. This was an incredibly important step in the field: Since an analysis based on scoring non-metric traits is the most feasible approach for characterizing dental traits, a physical plaster model is not only a good way of establishing a visual reference and therefore reduce intraobserver and interobserver inaccuracies, but it is also a powerful learning tool for students in the field.
More recently, Turner II et al. (1991) developed the Arizona State University Dental Anthropology System (ASUDAS), which has been the main scoring system since its original publication. The traits used in this system were chosen because they are easily observable and persistent, meaning they provide relevant information about a population via trait frequency. Many of the ASUDAS traits are invariable throughout hominoid evolution, suggested by Irish and Guatelli-Steinberg (2003), so they can also be used in characterizing non-human primates and early hominins dental morphology (Irish, 2013).
TEETH WEAR AND DENTAL PATHOLOGIES
As discussed before, enamel is not capable of remodelling after its maturation. With time, the forces applied by masticatory muscles on the occlusal/incisal surfaces of teeth cause the enamel to wear down. Patterns of wear can be used to access dietary behaviours. Hunter-gatherers may use their anterior teeth to process hides, causing the incisal surfaces to wear down faster. Early agriculturalists, on the other hand, wore down their posterior dentition faster, due to abrasive particles introduced by processing grains using grinding stones (Smith, 1984). If the process of tooth wear is slow, secondary dentine is produced by remaining odontoblasts in order to protect the pulp.
Another effect of the forces applied on teeth is the slow decrease in crown height, causing a remodelling of the alveolar process (the portion of the jaw which contains the teeth sockets). This results in the continuous eruption of teeth throughout life, eventually causing tooth loss due to lack of bone support (Hillson, 2008). A different cause of tooth loss is periodontitis: Short episodes of inflammation caused by plaque alternated with periods of recovery leading to bone reabsorption and reduction in height of the alveolar process.
Caries is also an important component in tooth loss, but in a different way. Although the inflammation in pulp and periapical tissues caused by caries does not cause the remodelling of bone tissue associated with the loss of teeth, the pain inflicted by it makes human intervention likely to happen in order to remove the carious tooth. Caries is a demineralization process, caused mainly by the accumulation of a specific type of bacteria (Streptoccocus mutans) of the dental plaque (Loesche, 1998). There is a strong correlation between the presence of caries and the increase of sugar consumption during the twentieth century (Thylstrup and Fejerskov, 1994). There is also noticeable decrease in decay rate of children as a result of sugar rationing in Japan and Norway during World War II. The main cause of caries is commonly regarded as sucrose, but the similarity in the molecular structures of simple carbohydrates reflects a small difference in the cariogenicity of fructose, glucose, lactose and sucrose (Hillson, 2008).
The nature of enamel deposition is used in the context of forensic dentistry in various ways.(1) Teeth found in isolation may be matched comparing the brown striae and cross striations to determine the identity of an individual, as the whole dentition of an individual presents, roughly, the same pattern; (2) Historical stressful events (e.g. volcanic eruptions or long periods without food) are able to be matched biologically, if all individuals s dated to a specific period have similar disruption markers. (3) The first brown stria of Retzius, known as Neonatal line and caused by the physiological stress of birth, can be used to determine if infants died before or after they were born, since prenatal enamel does not usually contain striae (FitzGerald and Rose, 2008). (4) In modern forensics and criminology, in situations where most tissues were destroyed, the extraction of DNA through the pulp and dentin is practical for identification, due to the high chemical and physical resilience of these dental tissues (Datta and Datta, 2012). (5) The mineralized cells present in dental calculus provide an important source of ancient DNA.
With the advance of PCR (polymerase chain reaction), it is feasible to use DNA amounts as miniscule as 1 ng are useful for identification purposes (Sweet, 2001). Dental tartar/calculus retains pathogenic cells, human cheek cells and dietary components such as plant particles, therefore it is useful in many contexts. Because the collection of calculus is a simple and non-destructive procedure, whereas the most common process of collecting DNA from skeletal remains requires powdering of a portion of teeth or bones, the extraction of DNA from calculus presents a major advantage. Plant particles in tartar are useful for recreating specific aspects of a population’s diet. For instance, starch particles can help understand the methods for cooking and processing food (Henry et al, 2009). A well-preserved dietary record may be used to establish environmental changes, changes in subsistence patterns and migration (Dudgeon and Trump, 2012). It is difficult to extract genetic material from pathogenic agents of ancient skeletal remains, since the amount of DNA and RNA present is very small even in highly infected individuals (Stone, 2008). When it is possible to obtain sufficient pathogenic material from PCR, however, it provides strong evidence for migration of populations and the origin and development of disease throughout human history.
CONCLUSION
The dentition and the characteristics of certain aspects of dental morphology are useful in many scientific fields (embryology, genetics, anthropology, palaeontology, forensic science, et cetera). If one wishes to acquire more in-depth information about dental anthropology, see Scott and Turner II (1991) and Hillson (1996); Mammalian teeth are explored thoroughly in Hillson (2005), the same for primate dentition in Swindler (2002).
REFERENCES
Bailey, S. E., Pilbrow, V. C., & Wood, B. A. (2004). Interobserver error involved in independent attempts to measure cusp base areas of Pan M1s. Journal of anatomy, 205(4), 323-331.
Benazzi, S., Coquerelle, M., Fiorenza, L., Bookstein, F., Katina, S., & Kullmer, O. (2011). Comparison of dental measurement systems for taxonomic assignment of first molars. American journal of physical anthropology, 144(3), 342-354.
Black, G.V. (1902). Descriptive anatomy of human teeth. White, Philadelphia.
Boyde A. (1964). Structure and development of mammalian enamel. Ph.D. Thesis, Department of Anatomy, London Hospital Medical College.
Bunn H.T, Domínguez-Rodrigo M, Pickering T.R. (2012) How meat made us human: archaeological evidence of the diet and foraging capabilities of early Pleistocene Homo in Eastern Africa. In: Lee-Thorp J, Katzenberg M.A, eds. Oxford Handbook of the Archaeology of Diet. Oxford: Oxford University. In press.
Butler, P.M. Molar cusp nomenclature and homology. In Development, Function and Evolution of Teeth, ed. P. M. Butler and K. A. Joysey. London: Academic Press, pp. 439-454.
Dahlberg A.A. (1956). Materials for the Establishment of Standards for Classification of Tooth Characteristics, Attributes and Techniques in Morphological Studies of the Dentition, Zoller Laboratory of Dental Anthropology, Chicago, University of Chicago..
Datta, P., Datta, S. S. (2012). Role of deoxyribonucleic acid technology in forensic dentistry. Journal of Forensic Dental Sciences, 4(1), 42–46. doi:10.4103/0975-1475.99165
Dudgeon, J.V. and Tromp, M. (2012). Diet, Geography and Drinking Water in Polynesia: Microfossil Research from Archaeological Human Dental Calculus, Rapa Nui (Easter Island). International Journal of Osteoarchaeology. Available at: http://onlinelibrary.wiley.com/doi/10.1002/oa.2249/full [Accessed 16th February 2015]
FitzGerald C. M. (1995). Tooth Crown Formation and the Variation of Enamel Microstructural Growth Markers in Modern Humans. Ph.D. Dissertation University of Cambridge.
FitzGerald C. M. (1998). Do enamel microstructures have regular time dependency? Conclusions from the literature and a large-scale study. Journal of Human Evolution 35:371–386.
Fitzgerald, C. M. and Rose, J. C. (2008). Reading Between the Lines: Dental Development and Subadult Age Assessment Using the Microstructural Growth Markers of Teeth, in Biological Anthropology of the Human Skeleton, Second Edition (eds M. A. Katzenberg and S. R. Saunders), John Wiley & Sons, Inc., Hoboken, NJ, USA.
Grine, F.E. and L.B. Martin. 1988. Enamel thickness and development in Australopithecus and Paranthropus. In Evolutionary history of the robust australopithecines (ed. F.E. Grine), pp. 3-42. New York, Aldine de Gruyter.
Hanihara K. (1961). Criteria for classification of crown characters of the human deciduous dentition. Journal of the Anthropological Society of Nippon 69, 27-45.
Hanihara, K., Tamada, M. and Tanaka, T. (1970). Quantitative analysis of the hypocone in the human upper molar. Journal of the Anthropological Society of Nippon 78, 200-207.
Hastings M.H., Loudon A.S. (2006). Metabolic regulation: fasting in the dark. Current Biolology 16: R254 –R255.
Henry, A.G., Hudson, H.F. and Piperno, D.R. (2009) ‘Changes in starch grain morphologies from cooking’. Journal of Archaeological Science. 36 (3), 915-922.
Hilson, S. W. (1996). Dental Anthropology. Cambridge: Cambridge University Press.
Hilson, S. W. (2005). Teeth. Cambridge: Cambridge University Press.
Hillson S. W. (2008). Defects of dental development in the enamel of the tooth crown, in Biological Anthropology of the Human Skeleton, Second Edition (eds M. A. Katzenberg and S. R. Saunders), John Wiley & Sons, Inc., Hoboken, NJ, USA.
Hrdlička, A. (1920). Shovel-shaped teeth. American Journal of Physical Anthropology 3, 429–465.
Irish J.D., Guatelli-Steinberg D. (2003). Ancient teeth and modern human origins: An expanded comparison of African Plio-Pleistocene and recent world dental samples. Journal of Human Evolution 45, 113–144.
Irish J.D., Guatelli-Steinberg D, Legge S.S., de Ruiter DJ, Berger L.R. (2013). Dental morphology and the phylogenetic “place” of Australopithecus sediba. Science 340(6129), 1233062.
Loesche W.J. (1996). Ch. 99: Microbiology of Dental Decay and Periodontal Disease. In Baron S, et al.Baron’s Medical Microbiology (4th ed.). University of Texas Medical Branch.
Phulari, R. G. S. (2013). Textbook of Dental Anatomy, Physiology and Occlusion. India: JP Brothers Medical Ltd.
Risnes, S. (1998). Growth tracks in dental enamel. Journal of Human Evolution, 35, 331–350.
Scott, G. R. (1991). Dental Anthropology. Encyclopedia of Human Biology 2: 789–804.
Scott, G. R. and C. G. Turner II (1997). The Anthropology of Modern Human Teeth: Dental Morphology and Its Variation in Recent Human Populations. Cambridge: Cambridge University Press.
Swindler, D. R. (2002), Primate Dentition: An Introduction to the Teeth of Non-human Primates, Cambridge: Cambridge University Press.
Smith, B. Holly (1984). Patterns of molar wear in hunter–gatherers and agriculturalists. American Journal of Physical Anthropology 63(1): 39-56.
Steele, D.G and Bramblett C.A. (1988). The anatomy and biology of the human skeleton. College Station, Texas: Texas A&M University Press.
Stone, A.C. (2008). DNA Analysis of Archaeological Remains, in Biological Anthropology of the Human Skeleton, Second Edition (eds M. A. Katzenberg and S. R. Saunders), John Wiley & Sons, Inc., Hoboken, NJ, USA.
Sweet D. (2001) Why a dentist for identification? Dental Clinics of North America. 45: 237-51.
Swindler, D. R. (2002). Primate Dentistry: an Introduction to the Teeth of Non-Human Primates. Cambridge: Cambridge University Press.
Teaford, M.F. (2007). What Do We Know and Not Know about Diet and Enamel Structure?, in Evolution of the Human Diet: The Known, the Unknown and the Unknowable. Ungar, P. (Ed.). Oxford, Oxford University Press.
Thylstrup A, Fejerskov O. (1994) Textbook of Clinical Cariology. Copenhagen: Munksgaard.
Turner II. C.G, Nichol C. and Scott G. (1991). Scoring Procedures for Key Morphological Traits of the Permanent Dentition: The Arizona State University Dental Anthropology System. In Kelly M. and Larsen C.S (eds) Advances in Dental Anthropology. Willey-Liss, New York, pp. 13-31.
Wrangham, R. (2009). Catching Fire: How Cooking Made Us Human. Basic Books, 2009.
Yamada, H., & Brown, T. (1988). Contours of maxillary molars studied in Australian Aboriginals. American journal of physical anthropology, 76(3), 399-407.